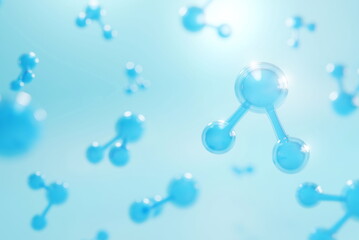
Mito ed energia
Prometheus and the chained molecoule
Human history is all about overcoming natural limits: we found the energy that forged the modern world, but by releasing carbon dioxide, we also warmed the globe. Capturing CO2 and re-injecting it back into the mining strata it came from is one possible solution.
11 min
P
rometheus, the god feared and loathed by his divine peers for his boundless love for mortals, embodies humanity’s perpetual struggle to transcend natural limitations. To find the myth that most accurately reflects our present challenge, we must journey back to the 5th century B.C. It was Prometheus, who bestowed fire upon mankind, symbolized the gift of technological and scientific power to transform the world. This immense power instilled fear among the gods.
Fire has been essential to human history. But, with all due respect to Aeschylus, it pales in comparison to its modern evolution: fossil fuels. The new Prometheus bears a symbolic name: Drake (Drago in Italian), the colonel who boasted of his title to secure the funds needed for drilling. In 1859, in Titusville, Pennsylvania, Drake provided humanity with rock oil, a fire of greater energy density, easier transportation, and remarkable adaptability. Like its close relatives coal and gas, oil is the result of a process spanning millions of years. This transformation occurs through the sedimentation of plant material in oxygen-free conditions and a very slow cooking process, ultimately forming hydrocarbons.
With the Drake Well (sometimes reality surpasses fiction), we unlocked access to a vast energy storehouse and malleable molecular structures that could be transformed into new objects. In essence, we gained both the building materials and the energy necessary to reshape the world. This discovery marked a transition from flow energy (muscular and weather-dependent) to stock energy (underground and constant), shifting from an organic economy to a mining-based economy.
The unprecedented progress of “fossil” man
In less than two centuries, we broke all the chains that hindered humanity’s growth and progress. Statistics on human lifespan, nutrition, education, and individual wealth reached a tipping point at the turn of the nineteenth century. The dire predictions of Malthus, Jevons, Hubbert, and millenarian fears were regularly disproved, and growth became exponential.
Just 50 years ago, we were predicting a food crisis, resource depletion, and other calamities. Yet, nothing has prevented the doubling of the world’s population and a 1,000 percent increase in per capita GDP. With the stock of fossil fuels, we overcame the natural unpredictability of weather (sun, water, and wind), the industrial exploitation of the biosphere (timber and animals), and land-use competition. Many children who once would have died in infancy now survive and grow old; today, 37 out of every 1,000 children die in the first five years of life, compared to 150 in the early 1970s.
The great economic and health successes of our recent history have brought not only benefits but also complexities. We now face a new technological challenge, spurred by the rapid and extensive success of “fossil” mankind: the need to contain and progressively eliminate the carbon emissions that naturally result from using the vast mineral stock we are transforming to fuel modernity.
Hydrocarbons are molecular structures that must be broken down by heat to produce energy and materials. Hydrogen and carbon must be separated to unleash their full Promethean potential. However, carbon has a flaw: it dislikes solitude and readily bonds with other molecules. When carbon meets oxygen in the air, it forms a greenhouse gas (carbon dioxide), leading to the gradual warming of the atmosphere. While we have tapped into our energy stock, we are also reintroducing prehistoric carbon into circulation.
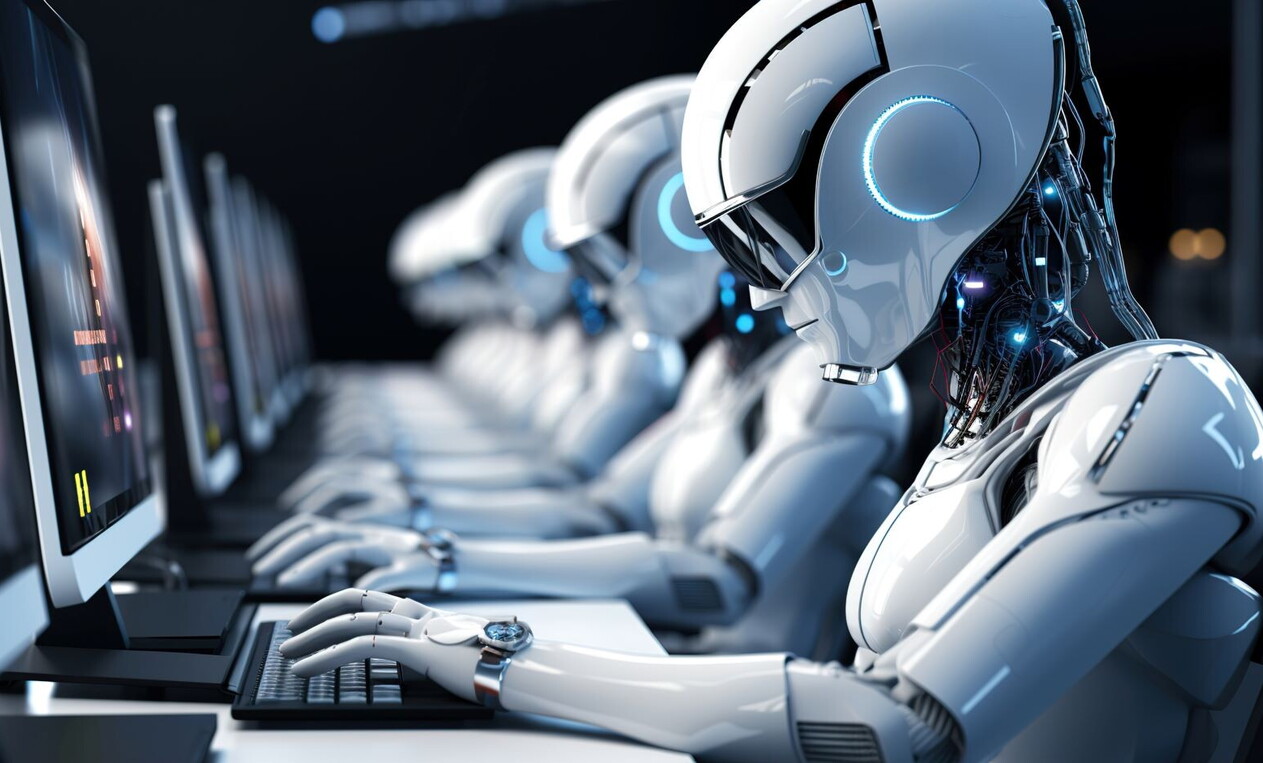
The obvious approach to curbing the increase in CO2 is to reduce our reliance on hydrocarbons by transitioning away from fossil fuels and increasing the use of alternative energy sources and electricity in final consumption. However, this is a limited option. Electrification of consumption faces challenges, such as the difficulty of using electricity for all our final needs (e.g., flying, sailing, smelting metals, or processing minerals and heating), storing energy produced through solar and wind power, and the low energy density of these sources, which necessitates massive land use.
In short, moving away from fossil fuels to rely on energy flow is costly, uncertain, and seasonal, requiring a radical change in our consumption systems that is not entirely feasible. The modern world is built on four pillars—steel, plastic, cement, and fertilizer—all of which have high-carbon industrial cycles upstream. Additionally, the increasingly dominant digital technology represents another carbon-intensive industrial process that will accompany us in the coming decades. New consumption areas, growing populations, and new industries will demand more objects, industrial processes, and emissions.
What’s more, the goal of rapid replacement conflicts with the differing priorities of development plans in emerging regions. These regions will continue to drive the demand for new materials and objects for many decades, inevitably relying on the industrial processes that underpin our civilization. Therefore, it is essential to explore alternatives to mere reduction in use, specifically the need to develop technologies that can harness carbon, store it, and potentially use it
Carbon capture
Storing a gas like CO2 is not a new activity. Since the 1930s, it has been used to enhance oil field productivity and manage fluctuations in gas consumption between summer and winter by reinjecting gas into depleted fields.
There are several reinjection and upstream capture techniques for CO2. Currently, these methods enable the absorption of about 45 million tons of CO2 per year from around thirty plants. While this number is negligible compared to the 37 billion tons emitted annually, these technologies were primarily used for other purposes, such as increasing oil recovery, and lacked a pricing system to justify broader application. However, with rising CO2 prices, the number of new projects in operation and under construction has surged in recent years, bringing the total estimated capacity to about 360 million tons per year from around 400 plants. This is a significant leap from just 50 million tons in 2019.
This is just the beginning of a massive industry. According to IPCC and IEA estimates, by 2030, we will need to capture and store 1 billion tons of CO2 per year, increasing to 10 billion by 2050. This is an inevitable business if we are to pursue a realistic transition path. Efficient CO2 capture and storage systems are essential to maintaining the industrial processes that provide us with necessary products and goods, including those required for the energy transition. Attempting to execute the energy transition without such systems would lead to decline and impoverishment.
Sanctioning a Carbon Capture and Storage (CCS) project is a complex issue. Both upstream capture activities at industrial facilities and all downstream collection and injection activities must be aligned, ensuring the entire process is regulated to account for investment risks and make it economically viable. In recent years, there has been growing awareness in the most emission-intensive industries, and projects have been analyzed to evaluate the cost structure. The cost of capture is the most significant element in the value chain, accounting for about two-thirds of the total cost. It is determined by the concentration of carbon dioxide in combustion processes, which varies by industry.
For example, in a thermal power plant, carbon dioxide concentration ranges from 10 percent in a coal-fired plant to 3-4 percent in a gas-fired plant. In a petrochemical plant, concentrations can reach about 10-15 percent, with peaks of 20 percent in steam reformers. Blast furnace steel plants see concentrations of 20-25 percent, and cement plants range between 20 and 30 percent. Consequently, the unit cost of CO2 in such activities ranges between USD 40 and USD 80 per ton. Capturing CO2 directly from the air presents a different challenge: the atmospheric concentration of CO2 is extremely low, at just 0.04 percent, making the costs still prohibitive.
In contrast, storage and transport have a relatively small impact on the cost structure and depend on the availability of existing infrastructure in depleted reservoirs. These costs can reasonably range between USD 20 and USD 30 per ton. In short, with the current emission trading system structure, we are already approaching the threshold of affordability for the most emission-intensive plants. However, some large industrial projects still require tariff support to ensure their viability.
The needs of future generations
Prometheus’s quest —humanity’s quest to transcend natural limits— continues to resonate all these centuries later. We have harnessed the molecules and energy that built the modern world, but in doing so, we have released so much carbon we have warmed the globe. One urgennt solution is to capture CO2 and return it to the earth, closing the loop. This strategy will allow us to utilize essential resources to meet the needs of future generations and support the artificial intelligence systems that will be integral to our future